Challenging Einstein
At a glance
- A key goal of the SKA telescopes is to test Einstein's General Theory of Relativity in extreme environments
- Astronomers will focus on environments where gravity is exceptionally strong, such as around supermassive black holes
- They will seek to indirectly detect gravitational waves - ripples in the fabric of space-time - using a cosmic network of rapidly spinning neutron stars, also called pulsars
- The SKA telescopes will detect tiny changes in the arrival time on earth of the signal emitted by millisecond pulsars, characteristic of a gravitational wave passing through the path of the signal
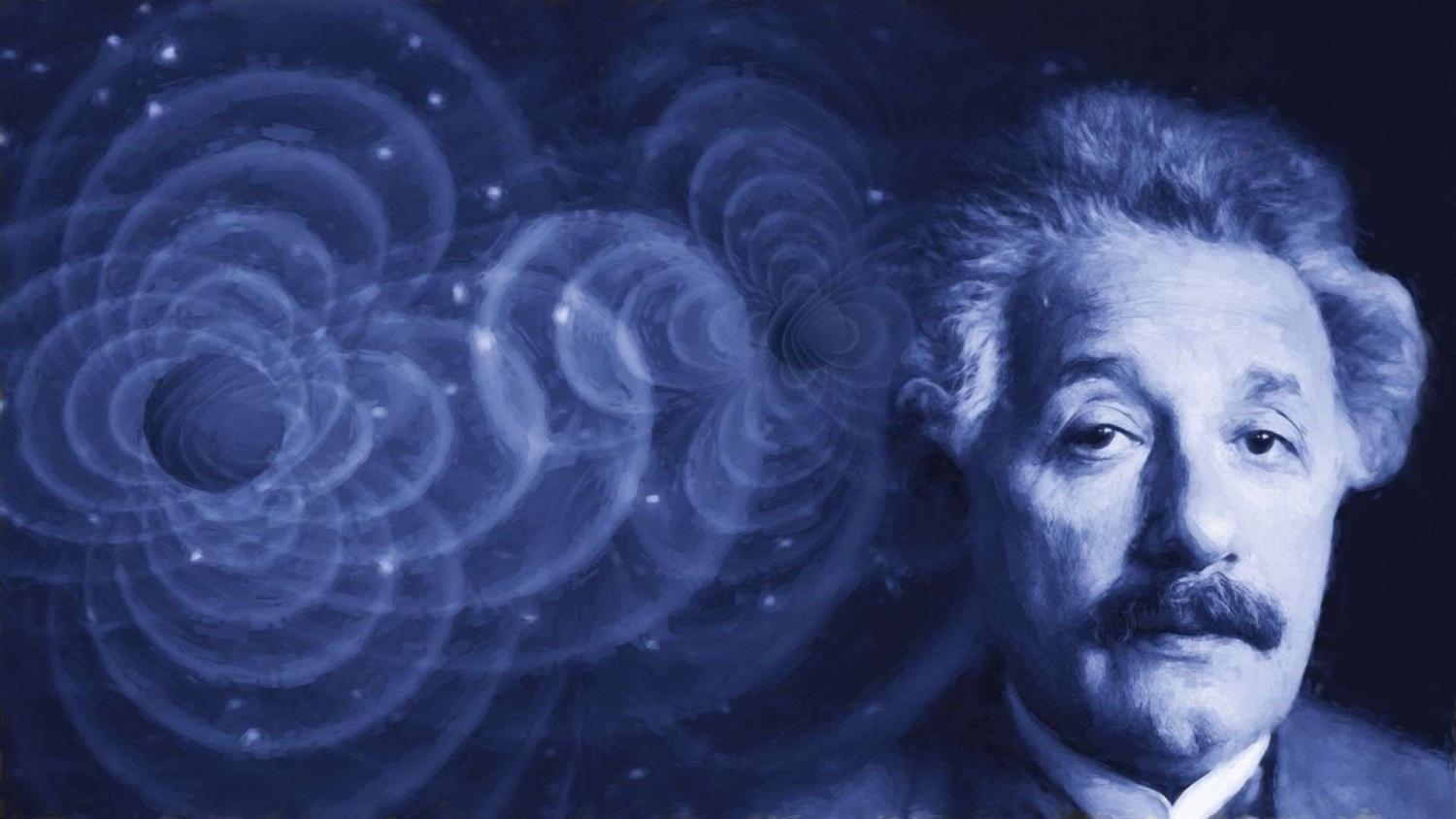
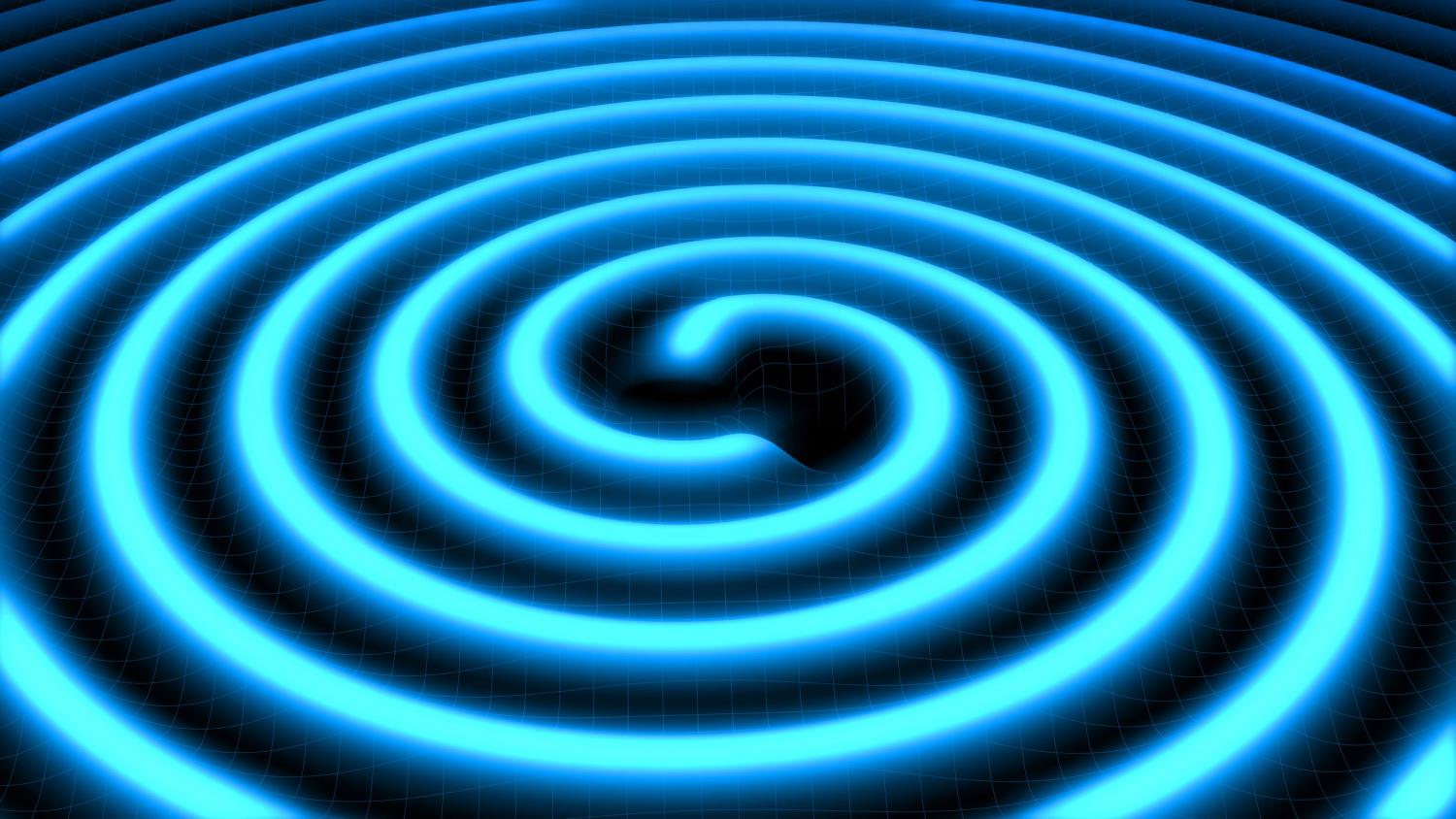
For more than a century, Albert Einstein’s General Theory of Relativity, which predicted the effects of curved space-time, has stood firm against every test that physicists have thrown at it. Now the dawn of the SKA era will bring about the biggest challenge yet.
The telescopes’ unrivalled capabilities will test Einstein’s theory in the most extreme conditions in the Universe, where gravity is far stronger than in our own Solar System, such as around black holes. They will also detect gravitational waves, ripples in the fabric of space-time which affect the underlying structure of the Universe, by observing their effect on extremely dense spinning objects called pulsars.
The fabric of our Universe isn’t like a flat sheet; it has distortions, or curves, caused by the mass of planets, stars and other objects. These curves in turn affect how matter moves through the Universe, which we perceive as gravity. The curvature involves time as well, with the gravitational effect of massive bodies like black holes slowing down time for anybody nearby. This is what Einstein detailed in his 1915 theory.
What remains untested is whether this theory holds true in all cases. By pushing beyond the weak gravitational field conditions of our Solar System and into strong-field environments, which may be extreme enough to show deviations from general relativity’s prediction, we can create the most thorough test yet undertaken.
Catching a gravitational wave
Einstein’s theory included predicting the existence of gravitational waves, ripples in space-time propagating at the speed of light as the result of the motion or collapse of massive objects. A century later, in 2015, the LIGO experiment directly detected gravitational waves for the first time.
These waves are particularly interesting for astronomers because telescopes are only sensitive to different kinds of light – different parts of the electromagnetic spectrum – emitted by objects (infrared, radio, optical light and so on), whereas gravitational waves affect the underlying structure of the Universe.
Gravitational waves – just like sea waves – come in different sizes depending on their origin. The SKA telescopes will detect some of the biggest, caused by enormous gravitational events. Both the birth of the Universe in the Big Bang and the collision of supermassive black holes in the centre of galaxies much later would be expected to produce gravitational waves that still propagate through our neighbourhood.
The SKA telescopes’ will detect these waves by measuring the distortion of time rather than space. How will they do it? By finding and measuring changes to pulsars, the collapsed spinning cores of dead stars.
Pulsars: reliable cosmic lighthouses
Their rapid and regular rotations make pulsars incredibly precise space clocks, as accurate as the best atomic clocks on Earth. They emit a pulse of radio waves like a lighthouse beam, which radio telescopes can detect from Earth. It is this accuracy, and the SKA's ability to detect even the most subtle variations in this caused by a passing gravitational wave, which will hopefully enable this breakthrough in science.
The SKA will be able to time millisecond pulsars (which are both faster and rarer than an average pulsar) typically to 100-nanosecond precision, and even better in some cases. This means it can predict a pulse’s arrival time to better than 10,000,000th of a second, a level of accuracy which is essential to spot the tiny deviations caused by a gravitational wave.
By timing a whole array of pulsars, the SKA will effectively create a cosmic observatory, tracking a gravitational wave as it passes through our galaxy.
Extreme astrophysics: gravity and black holes
Black holes are among the most extreme environments in the Universe, the remnants of massive stars which have gravitationally collapsed and exploded in a supernova. As incredibly dense objects with a huge mass, the gravitational fields around them are as strong as it gets - so strong that after a certain point, known as the event horizon, nothing can escape a black hole's grasp, not even light. This makes them an ideal place to test and refine Einstein’s theories on gravity to the absolute limit.
To do so, the SKA telescopes will also look for something which is expected to be very rare: a pulsar orbiting a black hole. The SKA telescopes’ unique sensitivity means we expect to be able to find a pulsar orbiting a black hole in the disk of our galaxy, and possibly around the super-massive black hole its centre.
Around black holes, the strength of gravity causes space-time to become extremely warped. On Earth, we would expect this warping to cause delays to the arrival times of signals (or pulses) from a pulsar, something the SKA telescopes will be able to spot with unprecedented precision. That delay would also be expected to vary depending on where the pulsar is in its orbit of the black hole, and how much space-time distortion it encounters as a result.
Einstein's theory included predictions about the nature of black holes and the delays they would cause to light travelling through space, so astronomers will aim to compare the theory with the actual measured reality - the ultimate test of General Relativity. If the two are consistent, Einstein wins again. If not, then we know there is something missing in our understanding, opening up a whole new area of astrophysics.