SKA-Low
Exploring the Universe from Australia
SKA-Low in numbers
Size: 131,072 log-periodic antennas spread between 512 stations
Collecting area: 419,000m2
Maximum distance between antenna stations: 74km
Frequency range: 50 MHz - 350 MHz
From a remote site in Western Australia's Murchison shire on the traditional lands of the Wajarri Yamaji, SKA-Low's 131,072 antennas will unlock one of the few remaining mysterious ages of the Universe.
The telescope will explore the first billion years, mapping the structure of the early Universe for the first time, watching the births and deaths of the first stars, and helping us to understand how the earliest galaxies formed.
SKA-Low's antennas are divided into 512 stations, with 256 antennas per station. From a central compact core measuring 1km across, further stations are distributed in three spiral arms radiating out from the centre across a huge area; the maximum distance between the two furthest stations will be 65km.
Standing at 2m tall, SKA-Low antennas are markedly different from the traditional "dish" style of SKA-Mid, because at low frequencies this type of "wire" antenna is much more efficient. These deceptively simple-looking structures are combined with state-of-the-art back-end technologies which make them incredibly powerful.
Institutions in Australia, China, Italy, Malta, the Netherlands and the UK contributed to the design of SKA-Low, and its many components will be manufactured all over the world before being shipped to Western Australia for construction at the Murchison Radio-astronomy Observatory.
How does it work?
SKA-Low is a "mathematical" telescope that works by filtering out what is not desired from the observable sky. Its antennas see the whole sky, and through data processing astronomers can "point" in different directions even though the antennas have no moving parts.
The antennas are made of horizontal branches of different lengths, called dipoles. From top to bottom, pairs of dipoles get increasingly bigger. Each of them absorbs radio waves coming from the sky, and the bigger the dipole, the longer the wavelength it absorbs. When radio waves excite the dipoles an electrical current is generated, collected via a central transmission line and transmitted to the top of the antenna. Here a pair of low noise amplifiers boost the weak signal without adding unwanted interference.
The signal is then sent from the antenna to a smartbox located at each station, where the electrical signals are converted to optical signals for transmission over optical fibre at a rate of 7.2 Tb/s to the on-site Central Processing Facility (CPF). The more far-flung stations along the telescope’s spiral arms have remote processing facilities, each dealing with signals from six stations before sending them on to the CPF.
It is here that the mathematical telescope is truly realised. When signals arrive in the CPF they face a complex barrage of processing technology which sees them clean and digitised. They are then sent to an off-site processing centre, located in Perth, where the correlator and arrray-beamformer combine signals from multiple stations to make the telescope digitally “point” in one or multiple directions in the sky. SKA-Low’s pulsar search and timing engine can then search within these beams for pulsars and other transient phenomena, and time them accurately.
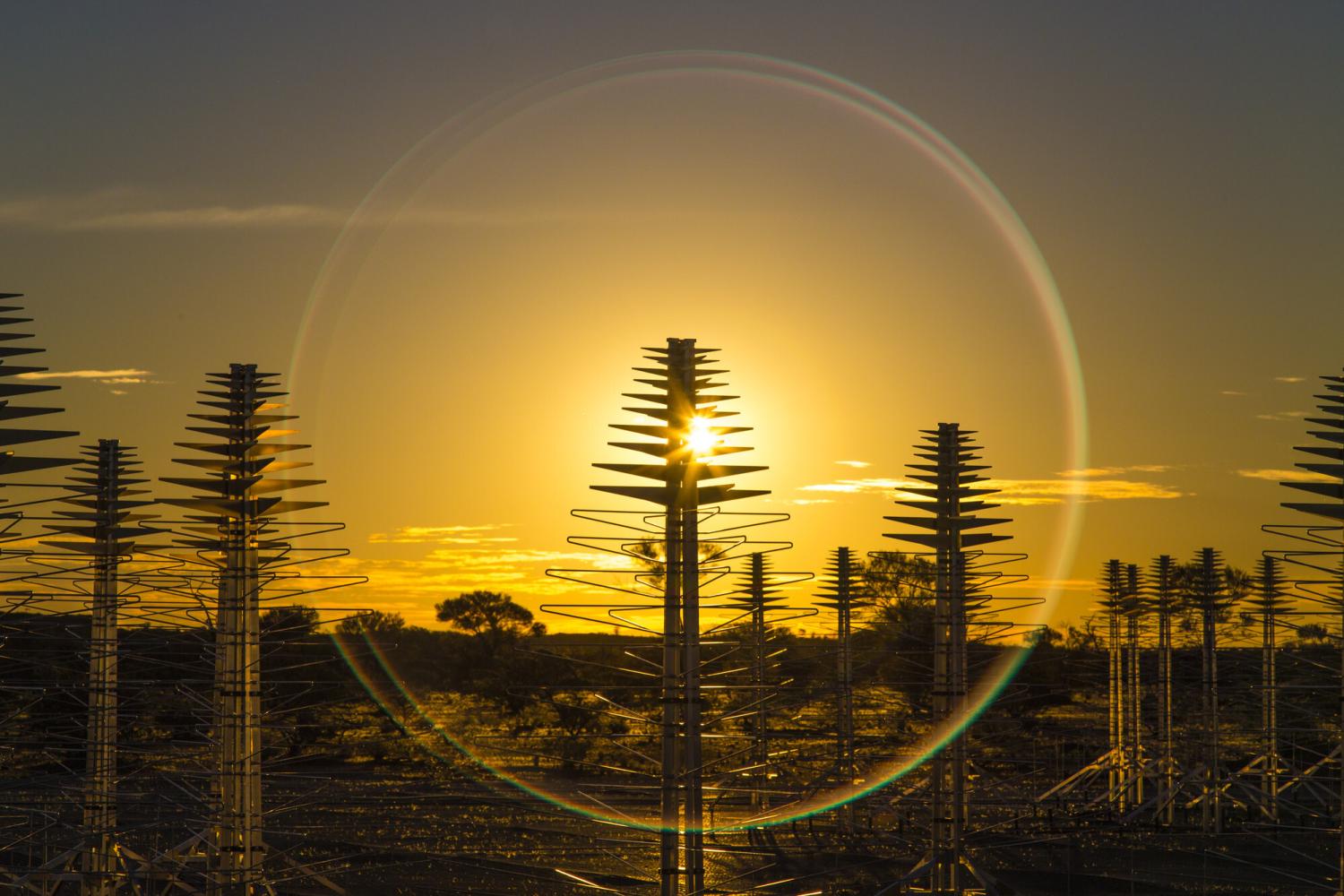
Revealing the sky's faintest details
The sheer scale of SKA-Low means that, compared to its predecessors, it will provide a massive increase in resolution, sensitivity and survey speed, three of the most important elements for radio telescopes.
Resolution: making the cosmos clearer
SKA-Low's 131,072 antennas will be configured with a dense central core of antennas, surrounded by three spiral arms; the longest distance (or baseline) between antennas will be 65km. As interferometers, both SKA telescopes' long baselines mean they can achieve higher resolution than a single large telescope, thanks to a technique called aperture synthesis. High resolution translates into sharper images which reveal much finer detail, a bit like putting on reading glasses to see things more clearly.
Sensitivity: shining a light on the distant Universe
SKA-Low is sensitive to low frequency radio signals; these are the very faintest signals, which have been travelling for billions of years and have been "stretched" to longer wavelengths by the expansion of the Universe. In radio astronomy, the sensitivity of a telescope depends on the collecting area available to capture signals from space. SKA-Low provides 400,000m2 of collecting area, meaning even the weakest of signals can be detected, combined and enhanced in a way that has never been possible before.
Survey speed
Carrying out large sky surveys requires a telescope with a wide field of view to study large areas of sky efficiently. Rather than physically moving the antennas, SKA-Low uses a technique called beamforming to digitally "point" the telescope anywhere in the sky by combine signals from some or all of the antennas in the array. As many different beams can be created at once, pointing all over the sky, this gives the telescope a huge field of view, producing much faster survey speeds than other telescopes.
How does SKA-Low compare?
Compared to the LOFAR telescope in the Netherlands, which is currently the best similar instrument in the world, SKA-Low will have 25% better resolution, eight times the sensitivity, and will be able to survey the sky 135 times faster.
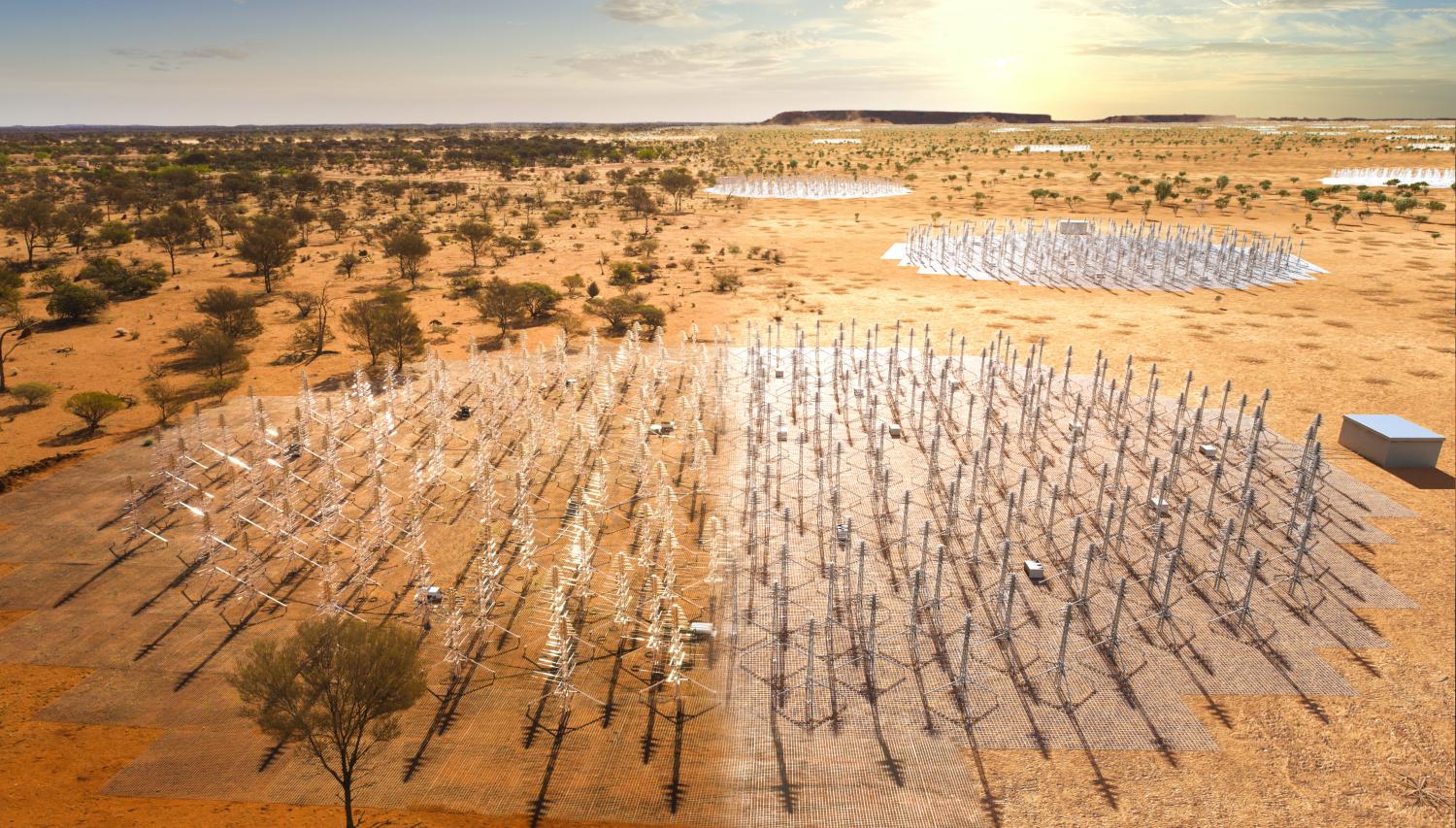
Why do the antennas resemble Christmas trees?
Traditional dipole antennas are limited in the frequency ranges they can receive, which is why the SKA-Low design uses log-periodic antennas which are constituted by multiple “triangular teeth/dipoles” to cover a wider range of frequency.
The bigger the dipoles, the lower the frequencies they can detect. For this reason, the dimension of the triangular teeth of the log periodic is gradually scaled to receive signals within the entire 50 MHz to 350 MHz frequency range. In particular, the longest and the shortest dipoles drive the lowest and the highest operating frequencies, respectively, while the rest of the dipoles contribute to receive the intermediate frequencies and to ensure that the antenna is working in a uniform way across the frequency range.
In this way, they can both detect the "oldest" signals from space which have been stretched to longer wavelengths (i.e. lower frequencies) due to the expansion of the Universe during their journey to Earth (a process called redshift), as well as the shorter wavelengths which originate (relatively) closer to Earth.
SKA-Low makes the most of building a very large array of very simple elements, generating the telescope’s performance by combining all those simple elements to reduce individual flaws. As a result of such a design, there are fewer single points of failure, and any failure (an antenna or a station being offline) has less of an impact on the overall performance.
Did you know?
Lower frequencies correspond to longer wavelengths.
The lowest frequencies are absorbed by the lowest dipoles on the antennas.
SKA-Low's antennas do not move, but can be digitally "pointed" in any direction through a process called beamforming.
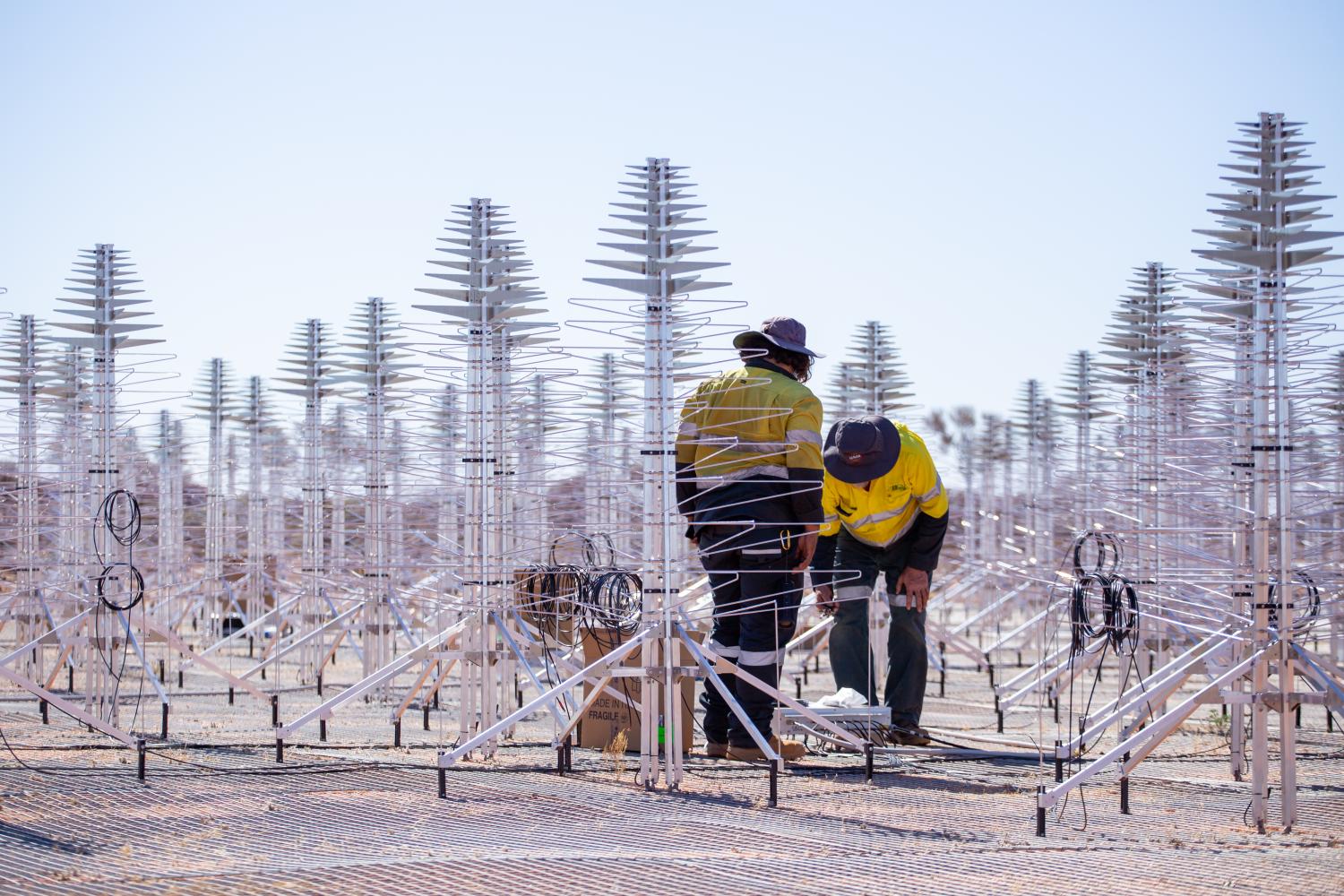
Design considerations
Major science endeavours are always a trade-off between scientific requirements and a range of other considerations, among them for SKA-Low: the feasibility of mass producing so many antennas, cost effectiveness, and ensuring the materials can withstand the extremes of the remote environment. Electrical components on each station, and on the site more widely, also have to be extremely well shielded to avoid the telescope disrupting its own observations with self-generated electrical interference.
The size of SKA-Low also demanded that the antenna design could be reliably replicated tens of thousands of times, and that it would be quick to install. These complex requirements led to several design refinements which resulted in the final antenna. Learn more about the process on our pre-construction design website.